KM Myrvold
The Lapwai Creek project
LET'S TAKE A STEP BACK
Ecology is, in its simplest form, the study of the distribution and abundance of organisms. It spans three levels of biological organization, from the individual organism, to the population, to the community. At the individual level, ecology deals with the relationship between the individual organism and its environment. At the population level, ecology concerns the distribution, abundance, trajectory of a particular species. There are two ways to study the population: as an entity with quantitative characteristics in its own right, or as a heterogeneous group made up by a combination of individuals with individual traits. Finally, community ecology concerns the structure of communities and the flow of energy, nutrients, and chemicals through them (Begon et al. 1996).
The unit for classification and management is most commonly the population, or some segment of a larger population, and most of the efforts by ecologists revolve around identifying which factors govern abundance and distribution. Populations are limited by external or environmental factors such as weather that act independently of population size, and regulated by density dependent factors, that is, when the per capita growth rate of the population depends on its own density (Sinclair and Pech 1996). Which of the two that is more important has been the focus of serious debate (Nicholson 1933, Andrewartha and Birch 1954). It has become clear that the debate is not a dichotomous one, as populations are governed by a combination of the two (Begon et al. 1996, Karels and Boonstra 2000, Leirs et al. 1997). Their relative importance varies by population in relation to their abiotic conditions, community organization, and the population’s size and –trajectory, all of which are dynamic in time and space.
In most cases, however, other factors than population processes and their interactions with the natural environment also govern the distribution and abundance of organisms. Global change (referred to as the sum of climate change, societal change, and land use changes) affects species abundance and distribution directly through alterations to their habitats and their management, and indirectly via alterations to the climate and nutrient cycles (Vitousek 1992, 1994, Parmesan and Yohe 2003, Thomas et al. 2004). These impacts can occur at almost any scale, and the effects on abundance and distribution depend on both the nature of the impacts as well as the life history characteristics of the species. Explicitly accounting for these factors in any ecological study is hence of great importance.
Although few biomes and ecosystems remain unimpacted by global change, some are more severely impacted than others. Freshwater ecosystems, large or small, flowing or still, are among the most altered ecosystems worldwide due to human dependence on water for consumption, power generation, transportation, and recreation (Carpenter et al. 1992, Dynesius and Nilsson 1994, Allan 1995, Poff and Zimmerman 2010). In the case of running water, dams and diversions alter the flow regime by means of the magnitude, frequency, duration, timing, and rate of change in streamwater flow, with the result that very few streams function as uninterrupted continua (Stanford and Ward 2001). This has important consequences for physical habitat configuration and temperature to mention some, and obviously these changes to the physical template affect the biological communities that can inhabit the stream.
The consequences of global change and flow alterations are particularly evident in anadromous salmonids. Pacific salmon and steelhead have disappeared from about 40% of their historical range in California, Oregon, Washington and Idaho over the last century, and remaining populations are often greatly reduced from historical levels (Nehlsen et al. 1991, Gustafson et al. 2007). The Columbia River has shown some of the most severe declines in salmon populations along the Pacific Coast, where steelhead and salmon numbers have declined dramatically since the 1870s. From a historical return of 8-10 million fish to the Columbia River alone, the annual returns are usually in the 10-20% range today (Chapman 1986), with the majority of the individuals having begun their lives in hatcheries (NRC 1996).
Anadromous salmonids have complex life cycles, and utilize a wide range of habitats throughout their life (Quinn 2005). Attributing the declines to any particular factor was therefore a challenge in early investigations, oftentimes confounded by remediation measures and limited by technological shortcomings. The early declines of anadromous salmonids in the Columbia River Basin have largely been attributed to overexploitation and habitat modification (Chapman 1986), and later to the construction of hydropower dams on the mainstem rivers, which impacted all upstream stocks (NRC 1996). It was not until the seminal paper by Nehlsen et al. (1991), however, that a unified approach to understanding the status and trajectories of Pacific salmon, and setting regulatory goals for their recovery, were initiated.
With the resultant growing concern over the effects of these changes and an increased willingness to protect and restore the remaining populations, several populations of salmon and steelhead in the Columbia River Basin were listed under the Endangered Species Act in 1997. Because multiple, often site-specific factors have contributed to the declines of all populations, a critical step on the road to recovery was to identify the ecological and anthropogenic factors by which each population is affected.
In addition to the importance of investigating the limiting factors for population viability, ecological studies in impacted systems provide an opportunity to study ecological processes and test ecological theory. The great plasticity of individual traits (DeAngelis and Mooij 2005), feedback and compensatory mechanisms in population regulation (Murdoch 1994, Sinclair and Pech 1996, Hixon et al. 2002), and relationships with the physical environment (MacArthur and Pianka 1966, Rosenzweig 1981, Gaillard et al. 2010) might not appear as evidently in populations that are at or close to their quasi equilibrium. However, following some change or perturbation to the population or its habitat, these very mechanisms allow for the population to rebound towards former size (Turchin 1999). Perturbations that create gradients of biotic response, such as varying densities, hence provide a good opportunity to study individual responses and population processes along the resultant density gradient.
One such situation is found in the Lapwai Creek watershed in North-Central Idaho, United States, where competing demands for stream water has caused declines in a population of steelhead, Oncorhynchus mykiss. The population was listed as threatened under the Endangered Species Act under the initial listing process in 1997, which prompted monitoring of the population trend and investigations of its limiting factors. This introduction reviews key aspects of the initiation of my research in the Lapwai Creek watershed by highlighting its societal, legal, and technical context. The purpose is to provide a thorough background and introduction to the population that is used as the study system. First, I will highlight the listing process of Snake River steelhead under the Endangered Species Act, and the actions that were prompted as a result of this determination. Secondly I will introduce the study system, the water diversion infrastructure, the potential impacts that the operations can have on flow and fish habitat, and the following Biological Opinion, which concern the effects of the operation on steelhead population viability.
SNAKE RIVER STEELHEAD
Due to the declines in salmonid abundances in general, and steelhead in particular, the National Marine Fisheries Service (NMFS) received in 1994 a petition to list steelhead throughout its range in California, Oregon, Washington, and Idaho under the Endangered Species Act (ESA). The Endangered Species Act establishes a national program to conserve threatened and endangered species of fish, wildlife, plants, and the habitat on which they depend. The ESA defines an endangered species as one that is in danger of extinction throughout all or a significant portion of its range, and a threatened species as one that is likely to become endangered in the foreseeable future throughout all or a significant portion of its range (ESA sections 3(6) and 3(20), respectively).
Section 4(a)(1) of the ESA and the listing regulations (50 CFR part 424) establish procedures for listing species. The Secretary of Commerce must determine, through the regulatory process, if a species is endangered or threatened based upon any one or a combination of the following factors: (1) The present or threatened destruction, modification, or curtailment of its habitat or range; (2) overutilization for commercial, recreational, scientific, or educational purposes; (3) disease or predation; (4) inadequacy of existing regulatory mechanisms; or (5) other natural or human-made factors affecting its continued existence.
In 1996, NMFS completed a comprehensive status review of West Coast steelhead populations in Washington, Oregon, Idaho, and California, and identified 15 Evolutionarily Significant Units (ESUs; see below) within this range. This resulted in a final rule to list two ESUs as endangered and three as threatened under the ESA on August 18, 1997 (NMFS 1997). Snake River steelhead was one of the threatened ESUs in that initial listing. Because of legal and other issues (see below), all listings were reaffirmed and/or revised on January 5, 2006 (NMFS 2006a). The 2006 listing included a total of nine threatened and one endangered ESUs of West Coast steelhead, some of which had previously been listed. Because the ESA warrants protection for the species as well as their habitat, critical habitat for 10 West Coast steelhead ESUs was designated by NMFS on September 2, 2005 (NMFS 2005). The rule identified 33.201 km of streams in the Pacific Northwest of importance to threatened or endangered anadromous salmonids, of which the Lapwai watershed was one of the designated areas for Snake River steelhead.
Only certain populations, or groups of populations that exhibit some common traits, known as distinct population segments or evolutionary significant units, were listed. Section 3 of the ESA defines “species” as including “any subspecies of fish or wildlife or plants, and any distinct population segment of any species of vertebrate fish or wildlife which interbreeds when mature.” A group of Pacific salmon populations is considered an “evolutionarily significant unit” (ESU) if it is substantially reproductively isolated from other conspecific populations, and it represents an important component in the evolutionary legacy of the biological species (NMFS 1991). Further, an ESU is considered to be a “distinct population segment” (and thus warranting protection as a species) under the ESA (NMFS 2006a). A group of organisms is discrete if it is “markedly separated from other populations of the same taxon as a consequence of physical, physiological, ecological, and behavioral factors.” Significance is measured with respect to the taxon (species or subspecies) (NMFS 2006a).
The initial listing in 1997 warranted protected status only for the anadromous form O. mykiss, or steelhead, and this decision was reaffirmed in 2006. NMFS noted that there were uncertainties about the relationship of resident and anadromous forms of O. mykiss, but concluded that the two forms are part of a single ESU where they have the opportunity to interbreed. The US Fish and Wildlife Service, which has the jurisdiction over resident O. mykiss, or rainbow trout, disagreed and advised against the inclusion of resident O. mykiss in the ESU. Following a public hearing on the topic (see review in NMFS 2006a), NMFS noted that the resident and anadromous forms are “markedly separated” due to “physical, physiological, ecological, and behavioral factors” (NMFS 2006a), hence warranting the delineation as separate DPSs.
Multiple drivers have contributed through numerous mechanisms to the decline of West Coast steelhead. Although all the categories identified in section 4(a)(1) of the ESA have contributed, NMFS identified destruction and modification of habitat (category 1), overutilization for recreational purposes (category 2), and other natural and human-made factors (category 5) as being the primary reasons for the decline of both West Coast steelhead and Snake River steelhead (NMFS 2006a).
Salmonid populations persist over time as a function of life history characteristics unique to that population and adjacent populations. ESUs typically behave as metapopulations, at least over evolutionary timescales, in that there is some level of gene flow between populations (e.g. through straying), which contributes to the long term persistence of those populations (Hanski 1999). This spatial structure complicates the assessment of extinction risk (NMFS 2011) because the resilience of a population is contingent not only on its own status, but also on the status of adjacent populations. Consequently, in planning and monitoring their recovery, the metapopulations structure has to be accounted for. NMFS adopted was a two-step risk assessment approach according to certain criteria, first at the population level and secondly at the ESU level, to assess the viability of each. The criteria, known as Viable Salmonid Population (VSP) criteria consist of abundance, growth rate/productivity, spatial structure, and diversity (McElhany et al. 2000). Individual populations are assessed according to the criteria, and ESUs are assessed as the sum of individual populations. These larger-scale issues include total number of viable populations, geographic distribution of these populations (to ensure inclusion of major life history types and to buffer the effects of regional catastrophes), and connectivity among these populations (to ensure appropriate levels of gene flow and recolonization potential in case of local extirpations) (McElhany et al. 2000).
Infrastructure owned by the federal government represents a main cause of declines of steelhead in the Columbia River Basin. Section 7(a)(2) of the ESA requires Federal agencies to consult with U.S. Fish and Wildlife Service or NMFS to ensure that their actions are not likely to jeopardize the continued existence of endangered or threatened species or adversely modify or destroy their designated critical habitats. Section 7(b)(4) requires the provision of an incidental take statement (ITS) that specifies the impact of any incidental taking and includes reasonable and prudent measures (RPMs) to minimize such impacts (NMFS 2010).
Snake River steelhead constitutes an ESU and a DPS that occupies the Snake River Basin of southeast Washington, northeast Oregon and Idaho. Although the physical characteristics vary greatly over this range, the Snake River flows through terrain that is warmer and drier on an annual basis than the upper Columbia Basin or other drainages to the north (NMFS 1997).
Geologically, the land forms are older and much more eroded than most other steelhead habitat. The eastern portion of the basin flows out of the granitic geological unit known as the Idaho Batholith. The western Snake River Basin drains sedimentary and volcanic soils of the Blue Mountains complex. Collectively, the environmental factors of the Snake River Basin result in water that is warmer and more turbid, with higher pH and alkalinity, than is found elsewhere in the range of inland steelhead. As most inland steelhead Snake River steelhead are summer steelhead which enter fresh water from June to October and spawn in the following spring from March to May (NMFS 1997).
The Snake River steelhead Distinct Population Segment consists of six major population groups (MPG), characterized by major river basins, and 24 independent populations, representing groups of tributary drainages or distinct areas within each basin (Figure 1).
Figure 1. The map shows the six major population groups of Snake River steelhead, and their location within the Columbia River Basin (insert). The Lapwai Creek watershed is part of the Clearwater River Major Population Group. Adopted from NMFS (2010, NOAA Northwest Fisheries Science Center).
Lapwai Creek belongs to the Clearwater Lower Mainstem population of the Clearwater River major population group. Although the Clearwater Lower Mainstem is referred to as a population under the VSP criteria, I will refer to Lapwai Creek as a population in the following. The Clearwater Lower Mainstem consists entirely of A-run steelhead, which is found in relatively small tributaries such as Lapwai, Potlatch and Big Canyon creeks (NMFS 2005). The Lapwai Creek watershed is the westernmost and lowest elevation population in the entire major population group, and represents by such an important spatial diversity component.
Section 4(c)(2) of the ESA directs the review of the listing classification of threatened and endangered species at least once every five years to determine if any species should be 1) removed from the list; 2) have its status changed from threatened to endangered; or 3) have its status changed from endangered to threatened. The five year review following the 2006 reaffirmation concluded that Snake River steelhead had not met recovery criteria (NMFS 2011).
In their status analysis of the Clearwater River major population group, NMFS concluded there is “insufficient data on natural spawning abundance to determine productivity for the five populations in this MPG. The overall abundance and productivity risk rating therefore was identified as high for all populations, except for the Lower Mainstem Clearwater River which was identified as moderate” (NMFS 2011). Spatial structure and diversity risks currently are rated low for the Lower Mainstem Clearwater, Selway River, and the Lochsa River. The South Fork Clearwater River and Lolo Creek have moderate risk ratings for spatial structure and diversity. Four of the populations in the Clearwater MPG have an overall viability rating of high risk (South Fork Clearwater, Lolo Creek, Selway River and Lochsa River). The Lower Mainstem Clearwater River has an uncertain overall viability rating of “maintained”, referring to an extinction risk of 6 to 25 % in 100 years. Therefore, due to these population viability ratings, the Clearwater MPG is not viable and remains listed as threatened under the ESA (NMFS 2011).
In summary, West Coast steelhead and salmon have declined in numbers, distribution, and genetic composition due to a number of factors over the last century. Most notably, overfishing, and habitat destruction or -modification have been primary reasons for the declines. With growing concern over these declines, several distinct population segments of steelhead (corresponding to major river drainages) were listed under the Endangered Species Act between 1997 and 2006. Snake River steelhead was one of the population groups listed under the initial 1997 assessment. Major population groups within the Snake River segment share the common limitations of mainstem Snake and Columbia passage, but vary according to subbasin characteristics and population demographics. Further variation can be found within the major population groups at the scale of watersheds or drainages
LAPWAI CREEK AND THE LEWISTON ORCHARDS PROJECT
The Lapwai Creek watershed
The 694 km2 Lapwai watershed is situated southeast of Lewiston in North-Central Idaho. There are four major tributaries in the watershed that together form the 4th order Lapwai Creek which empties into the Lower Clearwater River, 237 m.a.s.l. The streams drain the north slopes of Craig Mountain (1530 m.a.s.l.) and carve steep canyons through the landscape. The predominant geology in the watershed is Columbia River basalt, with a band of Idaho Batholith in the upper, high elevation portion. The plateau above the escarpment is overlain with loess, and the predominant land use is dry land grain crops, which covers 34% of the entire watershed. Coniferous forests cover 29%, primarily at higher elevations above the prairie, and grasslands dominate the steep canyon sides and valley floors. The grasslands are utilized primarily for livestock grazing. Less than 5 % of the area is classified as developed for housing and infrastructure (Homer et al. 2007).
Because the Lewiston Orchards irrigation project was initiated over a century ago (see below), and logging, grazing, and agriculture have been widespread for even longer, little or no information is available on the hydrology prior to settlement. From a natural baseline it is however characteristic that runoff can be very flashy due to the local climate and the topography. First, mean annual precipitation is 490 mm, with higher amounts falling at higher elevations, and most of it in winter and spring. With the interior Columbia Basin climate of relatively rapid temperature fluctuations around freezing in winter, rain-on-snow events can cause rapid snowmelt and flashy runoff. Secondly, the catchments are high elevation and large relative to the steep and confined canyons through which they drain, with naturally limited meandering. The volume and velocity of the water during flooding by such has great capacity and competence to transport material downstream.
Historically, Craig Mountain has acted as a reservoir of water stored as snow, which gradually melted off during spring and early summer. In the recent century regional shifts in precipitation regimes and decreased foliage and forest cover due to logging have changed the runoff patterns. First, as with other interior Columbia drainages, regional climate change patterns have changed the precipitation regime in the elevation ranges found in the Lapwai watershed from a snow-dominated to a rain-dominated regime (Mote et al. 2003, Barnett et al. 2004). Secondly, logging and grazing practices on Craig Mountain have reduced the forest cover and structure. In turn, this leads to earlier and faster snow melt once temperatures are above freezing in late winter (Mote et al. 2003).
The stream gradients follow a shallow gradient on the plateau, followed by high gradients in the confined reaches below the escarpment. The stream gradients are lower below the confluences among the tributaries, but flood control levees and infrastructure (roads and railroad) have channelized the lower tributary and mainstem reaches (Richardson and Rasmussen 2007) and prevent connectivity with the floodplain (Williams 2011). The simplification of the stream channels has led to increased bed erosion and channel incision where the streams are confined and heavy deposition of gravel in unconfined reaches. During periods of low discharge, the widened channels resulting from the lack of channel forming processes exacerbate heat transfer and limit potential pool habitats (Poole and Berman 2001). The substrate is highly mobile in the lower reaches where deposition occurs, with most of it being cobble sized.
Although habitat quality in the Clearwater River Lower Mainstem Area is “largely in poor or non-functioning condition, or in streams with naturally low potential due to steepness or high water temperature”, and common problems limiting fish production have been attributed to “high summer temperatures, low summer discharge, flashy response to runoff, and moderate to severely degraded channels” (NMFS 2006b), anecdotal evidence of stranded fish during a flash flood, and operation of a traditional steelhead snag fishery on Mission Creek (Johnson and Stangl 2000) suggest that streams in the Lapwai Creek watershed produced larger numbers of anadromous fish in the past (NMFS 2006b). Sweetwater Creek is fed by Lake Waha through groundwater seeps at the Twenty One Ranch spring, which made for a steady supply of cool temperature water. Lake Waha was formed by a slope failure which filled in the canyon of the West Fork Sweetwater Creek with rock and soil material. There are no natural outlets except through the seeps. The stable temperatures likely created favorable conditions for steelhead by buffering against high temperatures in summer and low temperatures in winter (NMFS 2010). NMFS (2010) concluded in the environmental baseline that “The Lapwai Creek drainage has high potential for steelhead production if degraded habitats were restored, and it is an important source of A-run steelhead production”.
Water diversions and canal infrastructure
Located within the Lapwai Creek watershed is the Lewiston Orchards Project (LOP), which consists of a series of water storage reservoirs, diversion dams, and canals that provide irrigation water to the town of Lewiston. The diversion system was established as a private enterprise in 1906 to provide a steady water supply for domestic use and food production during the hot and dry summer months. The initial irrigation system provided a timber flume and a canal to carry water from Sweetwater Creek to Reservoir A (Mann Lake), but was later expanded (see below). Due to limited longevity of the early wooden flumes, extensive and frequent repairs were necessary to limit water loss. In 1939, the Irrigation District, aided by the Works Projects Administration, launched a program for replacing the wooden flumes with concrete bench flumes. To ensure reliable water supply irrigation and industry, the LOP was authorized in 1946 (60 Stat. 717, Public Law 79-569), and in 1948 the irrigation district deeded the entire collection, reservoir and distribution system, and the water rights, to BOR.
The diversion system captures much of the water that would naturally be feeding Webb and Sweetwater Creeks, the two westernmost streams in the Lapwai Creek watershed. The project facilities include four diversion structures (Webb Creek, West Fork Sweetwater Creek, Sweetwater, and Captain John Creek), feeder canals, three small storage reservoirs (Soldiers Meadow, Reservoir A (Mann Lake), and Lake Waha), a domestic water system including a water filtration plant which is no longer in use, and a system for distribution of irrigation water (Figure 2). The current use of the diverted water is for irrigation purposes, primarily of suburban residential land use, covering some 15.8 km2 of the area known as Lewiston Orchards (BOR 2013).
Water from the Webb Creek and Captain John Creek drainages is collected at Soldiers Meadow Reservoir. This water is released, when needed, and diverted into Sweetwater Creek by the Webb Creek Diversion Dam and Webb Creek Canal. Water from the West Fork Sweetwater Creek is stored in the offstream Lake Waha, which is fed by Lake Waha Feeder Canal. The stored water is pumped from Lake Waha back into the creek during the irrigation season. The water taken for irrigation purposes is diverted from Sweetwater Creek into Sweetwater Canal, which empties into Reservoir A. The system has the capacity to divert 0.91 m3s-1 (32 cfs) through the Sweetwater Canal, and 0.57 m3s-1 (20 cfs) through the Webb Canal (BOR 2009), and is operated from February through October.
Figure 2. Overview of the diversion and water storage system of the Lewiston Orchards Project. From NMFS (2010).
Effects of the water diversion system on flow and fish habitat
The diversion system directly affects flow and access to habitat in both the Lapwai and Captain John watersheds. For the Lapwai Creek watershed, surface flow is impacted most directly in Webb and Sweetwater creeks, and to a lesser extent in mainstem Lapwai Creek below the Sweetwater confluence. Flows in over 8 miles of Sweetwater Creek, 9 or more miles in Webb, and over 6 miles in Lapwai Creek within anadromous reach are impacted during the irrigation season. Further, the diversions presumably preclude upstream passage to another 11 miles of stream that contain resident O. mykiss (Chandler and Parot 2003, NMFS 2010), which means that this section could likely support anadromous O. mykiss as well. Captain John Creek is affected by the diversion of water from an intermittent headwater tributary which diverts water into the Webb Creek drainage. The lower reaches of Captain John Creek, which empties into the Snake River, serve as spawning and rearing habitat for steelhead and Chinook salmon. It is designated as critical habitat for these two ESUs, and as Essential fish habitat for Chinook and coho salmon, but the effects are smaller than in Lapwai Creek watershed.
Prior to 2006, LOID and BOR were allowed unrestricted withdrawal of water from Sweetwater, Webb and Captain John creeks. The timing and amounts varied widely from year to year depending on the amount of precipitation, air temperature, runoff, and demand for irrigation and domestic water (NMFS 2010). Streamflows below the diversions depended on the influx of groundwater and the availability of surface water in excess of what was diverted. It is likely that the operations have eliminated most rearing habitat in Webb and Sweetwater, and to a lesser degree in Lapwai below the Sweetwater confluence, by drying the streambeds in summer (NMFS 2010). The duration, extent, and timing of the drying likely varied from year to year according to the precipitation and runoff regime. When the LOP diverted all surface flows the usable habitat were found largely as disconnected pools and in reaches where groundwater surfaced. Collectively, this habitat had poor quality.
With the process of minimizing take of steelhead (see below), minimum flow requirements were established, which require LOID and BOR to spill a minimum amount of water past the diversions during the irrigation season. The streams are however still affected as the amount of water is different than what would be the case in absence of the operations.
An action area is defined under the ESA as “all areas to be affected directly or indirectly by the Federal action and not merely the immediate area involved in the action” (50 CFR Part 402). The affected streams include designated critical habitat for the Snake River Basin Steelhead DPS, and are designated as Essential Fish Habitat (EFH) for Chinook salmon and coho salmon. Based on this, NMFS (2010) defined the action area to “…consists of the following streams: (1) Captain John Creek from the headwaters of the North Fork to its mouth; (2) all portions of the Webb and Sweetwater Creek drainage systems where flows are altered by the LOP; (3) Lapwai Creek from its confluence with Sweetwater Creek, downstream to its mouth at the Clearwater River, and (4) the mainstems of the Clearwater River downstream of Lapwai Creek, the Snake River downstream of Captain John Creek to its confluence with the Columbia River, and the Columbia River downstream of the Snake River to the Pacific Ocean”.
Flow is the primary factor affected by the LOP, but the diversions also affect other physical variables that in turn affect channel forming processes. A channel is in equilibrium when the amount of sediment delivered to the channel equals the amount transported out. Both the delivery of sediments and the stream’s capacity to transport sediments are factors that matter in this equation, both of which are affected by the LOP. First, sediments are trapped behind the diversion dams, leading to excess scour downstream of the diversions. Secondly, lower discharge below the diversions decreases the stream’s ability to transport sediments. In sum these two factors can offset each other to some extent under conditions typically encountered in the irrigation season and when looking at the stream as a whole.
In summary, from a natural baseline the Lapwai Creek watershed spans a wide physiographic gradient despite its limited size. Compared to other systems in the Clearwater Lower Mainstem, the streams in Lapwai provide cool temperatures due to a relatively high elevation watershed and groundwater seeps. The low-elevation reaches of the tributaries and the mainstem Lapwai Creek are confined by infrastructures, and upland land uses largely affect runoff regimes and sediment and nutrient inputs to all the tributaries. Further, the two westernmost streams in the watershed have altered flow regimes due to water diversions. Because there is little or no data on the flow, habitat, or steelhead from the period before the operations started, it is impossible to know what conditions would be like in absence of the LOP. However, because the LOP withdraws water from critical habitat for ESA listed Snake River steelhead, the dam operator and owner (BOR) was required to quantify the impacts of the continued operations on steelhead population viability.
Biological Opinion of the future operation of the diversion system
Following the listing of Snake River steelhead under the Endangered Species Act in 1997, BOR initiated consultation with NMFS in 1998 at BOR facilities in the Snake River Basin upstream of Lower Granite Dam. BOR owns the LOP diversion infrastructure in the Lapwai watershed, which hosts listed Snake River steelhead and is designated critical habitat and Essential Fish Habitat. Each federal agency has an obligation to ensure that any action it authorizes, funds, or carries out is not likely to jeopardize the continued existence of any endangered or threatened species or destroy or adversely modify its critical habitat unless that activity is exempted (50 CFR 402.03). In short, the process consists of a Biological Assessment developed by the entity responsible for potential harm to reduce the impact, and a Biological Opinion of that assessment by the responsible authority. Under regulation 50 CFR 402.12(f), the “contents of a biological assessment are at the discretion of the federal agency and will depend on the nature of the federal action”.
The “nature of the federal action” in this case is the continued operation of the LOP, which is also referred to as the proposed action. Proposed actions are defined as “all activities or programs of any kind authorized, funded, or carried out…by Federal agencies” (50 CFR 402.02). Because the project withdraws water from streams that are designated as critical habitat for a listed species, and because BOR is a federal entity, BOR was required to consult with NMFS under section 7 of the Endangered Species Act and section 305(b)(2) of the Magnuson-Stevens Fishery and Conservation Act (MSA) to minimize the impact of the operation of the project on steelhead. With the Biological Assessment, the BOR formally requested NMFS, the authority with jurisdiction over federally listed species, to give a Biological Opinion of the proposed action. Section 7(b)(4) requires the provision of an incidental take statement that specifies the impact of any incidental taking and includes reasonable and prudent measures to minimize such impacts (NMFS 2010).
The Magnuson-Stevens Fishery and Conservation Act warrants protection for designated Essential Fish Habitat. The Pacific Fishery Management Council (1999) designated essential fish habitat for Chinook and coho salmon in Idaho, and the LOP action area falls within this zone. Because this dissertation focuses on steelhead, I will not review the MSA process in much detail here. In conclusion, NMFS (2006, 2010) found that Essential Fish Habitat in the Clearwater River, Snake River, Columbia River, and Captain John Creek is not adversely affected by the proposed action. Chinook and coho salmon were deemed adversely affected within the Lapwai Creek part of the action area (i.e. excluding Captain John Creek); coho through the same mechanisms as steelhead, and Chinook as described in the BiOp (NMFS 2010). Note that although the conservation measures under the MSA are similar to, they are not identical to those set forth under the ESA.
The following consultation history is long and will not be reviewed in great depth here. Instead, the reader is referred to NMFS (2006:2-6, 2010:3-8) for details. The process was concluded with a revised Biological Assessment (BOR 2009) in which the key scientific and operational criteria had been discussed by the parties involved. The main points of relevance for streamflow were 1) Collection of field data along Sweetwater and Webb Creeks, 2) Finalization of ramping rates, sediment removal, and gravel replacement plan, 3) Development of hydrologic and operational analysis tools to test the feasibility, reliability, and potential tradeoffs associated with candidate flow regimes, and 4) Provisions for additional summertime flows under given hydrologic and storage conditions. The BOR proposed to spill all water in the months of November to January as the irrigation canals are not operated during the winter. For the months of February to April, the minimum flow provided for Sweetwater is 7.8 cfs and 4.0 cfs for Webb. For the month of May the rates are 3 and 1.5, respectively, and for June through October the rates are 2.5 and 1.0, respectively.
In response to this, NMFS concluded that the proposed action was not likely to jeopardize the continued existence of steelhead in the Lapwai Basin and beyond, or result in the destruction or adverse modification of designated critical habitat for listed species in the same area (NMFS 2010). Although continued persistence was not deemed jeopardized, the action was expected to cause incidental take of steelhead. Section 9(a)(1) of the ESA prohibits the taking of endangered species without a specific permit or exemption, and section 4(d) extends the prohibition to threatened species such as Snake River steelhead. Taking is defined as “an action that harasses, wounds, or kills an individual of a listed species or harms a species by altering habitat in a way that significantly impairs its essential behavioral patterns” (50 CFR 222.102). Incidental take refers to takings that result from, but are not the purpose of, carrying out an otherwise lawful activity conducted by the Federal agency or applicant (50 CFR 402.02). Adult fish use the streams in the action area primarily during high flows and/or prior to the operation of the diversion canals, and the LOP is hence not likely to cause any incidental take of adult steelhead. NMFS further stated that “When the proposed action provides flows that are lower than those that would occur in the absence of the LOP, incidental take is likely to occur under the following circumstances: (1) Steelhead embryos and alevins may be killed when reduced surface flows either dewater redds or cause insufficient intergravel flows during the incubation period through the time when alevins would emerge from the gravels; and (2) stream flows artificially constrain steelhead abundance, growth, or survival due to reduced carrying capacity, high water temperatures or other stresses caused by flow reductions” (NMFS 2010). The incidental take exempted by this statement is the loss of steelhead from these two circumstances.
Directly quantifying the take resulting from the action is impossible, and hence NMFS relied on habitat indicators that were related to the mechanism of take. There are critical uncertainties about the effects of the operation. Although several potential mechanisms occur, they can be not be readily distinguished from the natural baseline (i.e. in absence of the operations), be directly attributed to the operation, or remain infeasible for monitoring purposes. The Biological Opinion discussed the shortcomings of relying on various parameters (water temperature, fish density) instead of others (flow, connectivity), and concluded that flow would be the best quantifiable habitat parameter, as low flows are the primary cause of take in the action area.
Because the action has the potential to take steelhead, BOR was required to implement certain reasonable and prudent measures. Reasonable and prudent measures are non-discretionary measures to minimize the take for the take exemption under section 7(o)(2). The 2006 Biological Opinion identified seven reasonable and prudent measures that were required to meet the incidental take statement, of which point 3, “the BOR shall monitor stream flows and any ancillary biological indicators necessary to determine if the extent of take exempted by this Incidental Take Statement is exceeded” (NMFS 2006) was the most important for the recovery monitoring.
In summary, because the LOP takes water from critical habitat for a listed species, the BOR was required to come up with a plan to minimize the impacts. BOR consulted with NMFS which has authority over anadromous salmonids. The technical and scientific discussion was concluded after 8 years, and resulted in several measures to minimize impacts. Among these measures, minimum flow requirements were the most important, and close monitoring of the effects were mandated.
PROBLEM STATEMENT
Identifying the limiting factors is of primary importance when setting goals for recovery and planning remediation measures to ensure viability of wild fish populations. As comes clear from the above review of the listing process, the key is to understand the mechanisms by which populations are governed and the conditions under which they are operant. At the same time as solving the applied problem, we can also use the effort to learn something of fundamental value to ecology, hence making an applied solution applicable to other systems.
The primary goal of this research was to identify mechanistic linkages between individual- and population-level demographic parameters and their environment, and to understand how population processes operate to shape individual performance. The study concerns primarily the abiotic and biotic conditions in the Lapwai watershed, where adults spawn and juveniles rear. These conditions are dynamic across space and time, meaning that their controls over individual performance and population demographic parameters vary in their absolute and relative importance.
This research does not directly cover the conditions encountered throughout the rest of the life cycle, such as mainstem migration, estuarine transitioning, and ocean migration and foraging. Although these phases are just as important for the overall persistence of an anadromous population, freshwater spawning and rearing habitat is a critical factor that controls the number of sea-migrating smolt. In addition, it is the only environment that the resident form of the species ever encounters, and the conditions can even influence the most central life history decision of residency or anadromy. To understand these ecological processes and the patterns they create, and making the findings applicable to other systems, we developed a series of studies to test ecological hypotheses. Several of these are made available under the list of publications.
REFERENCES
Allan, J. D. (1995) Stream ecology: Structure and function of running waters, Kluwer Academic Publishers, Dordrecht, The Netherlands.
Andrewartha, H. G. & Birch, L. C. (1954) The distribution and abundance of animals, University of Chicago Press, Chicago, IL.
Barnett, T., Malone, R., W., P., Stammer, D., Semtner, B. & Washington, W. (2004) The effects of climate change on water resources in the West: introduction and overview. Climatic change, 62, 1-11.
Begon, M., Harper, J. L. & Townsend, C. R. (1996) Ecology: individuals, populations and communities, Cambridge University Press, Cambridge, MA.
BOR (2001) Lewiston Orchards Irrigation District supplemental Biological Assessment. Bureau of Reclamation, Boise, ID.
BOR (2009) Biological assessment for operation of the Lewiston Orchards Project, Idaho. Bureau of Reclamation, Boise, ID.
Carpenter, S. R., Fisher, S. G., Grimm, N. B. & Kitchell, J. F. (1992) Global change and freshwater ecosystems. Annual Review of Ecology and Systematics, 23, 119-139.
Chandler, C. & Parot, R. (2003) Fish distribution and relative abundance of Big Canyon Creek, Lapwai Creek, Mission Creek, and Sweetwater Creek 2003. Nez Perce Tribe, Lapwai, ID.
Chapman, D. W. (1986) Salmon and steelhead abundance in the Columbia River in the nineteenth century. Transactions of the American Fisheries Society, 115, 662-670.
DeAngelis, D. L. & Mooij, W. M. (2005) Individual-based modeling of ecological and evolutionary processes. Annual Review of Ecology and Evolutionary Systematics, 36, 147-168.
Dynesius, M. & Nilsson, C. (1994) Fragmentation and flow regulation of river systems in the northern third of the world. Science, 266, 753-762.
Gaillard, J. M., Hebblewhite, M., Loison, A., Fuller, M., Powell, R., Basille, M. & Van Moorter, B. (2010) Habitat-performance relationships: finding the right metric at a given spatial scale. Philosophical Transactions of the Royal Society of London B, 365, 2255-2265.
Gustafson, R. G., Waples, R. S., Myers, J. M., Hard, J. J., Bryant, G. J., Johnson, O. W. & Weitkamp, L. A. (2007) Pacific salmon extinctions: quantifying lost and remaining diversity. Conservation Biology, 21, 1009-1020.
Hanski, I. (1999) Metapopulation ecology, Oxford University Press, Oxford, United Kingdom.
Hixon, M. A., Pacala, S. W. & Sandin, S. A. (2002) Population regulation: historical context and contemporary challenges of open vs. closed systems. Ecology, 83, 1490-1508.
Homer, C., Dewitz, J., Fry, J., Coan, M., Hossain, N., Larson, C., Herold, N., McKerrow, A., VanDriel, J. N. & Wickham, J. (2007) Completion of the 2001 National Land Cover Database for the conterminous United States. Photogrammetric Engineering and Remote Sensing, 73, 337-341.
Johnson, C. & Stangl, K. (2000) Clearwater River, North Fork Clearwater River, and Middle Fork Clearwater River Subbasins biological assessment of ongoing and proposed Bureau of Land Management activities on fall Chinook salmon, steelhead trout, bull trout, and BLM sensitive species. Bureau of Land Management, Cottonwood, ID.
Karels, T. J. & Boonstra, R. (2000) Concurrent density dependence and independence in populations of arctic ground squirrels. Nature, 408, 460-463.
Leirs, H., Stenseth, N. C., Nichols, J. D., Hines, J. E., Verhagen, R. & Verheyen, W. (1997) Stochastic seasonability and nonlinear density-dependent factors regulate population size in an African rodent. Nature, 389, 176-180.
MacArthur, R. H. & Pianka, E. R. (1966) On optimal use of a patchy environment. The American Naturalist, 100, 603-609.
McElhany, P., Rucklelshaus, M. H., Ford, M. J., Wainwright, T. C. & Bjorkstedt, E. P. (2000) Viable salmonid populations and the recovery of Evolutionary Significant Units. NOAA Technical Memorandum NMFS-NWFSC-42. Northwest Marine Fisheries Science Center, Seattle, WA.
Meehan, W. R. (1991) Influences of forest and rangeland management on salmonid fishes and their habitats, American Fisheries Society Special Publication 19, Bethesda, MD.
Mote, P. W., Parson, E. A., Hamlet, A. F., Keeton, W. S., Lettemaier, D., Mantua, N., Miles, E. L., Peterson, D. W., Peterson, D. L., Slaughter, R. & Snover, A. K. (2003) Preparing for climatic change: The water, salmon and forests of the Pacific Northwest. Climatic Change, 61, 45-88.
Murdoch, W. W. (1994) Population regulation in theory and practice. Ecology, 75, 271-287.
Nehlsen, W., Williams, J. E. & Lichatowich, J. A. (1991) Pacific salmon at the crossroads: stocks at risk from California, Oregon, Idaho, and Washington. Fisheries, 16, 4-21.
Nicholson, A. J. (1933) The balance of animal populations. Journal of Animal Ecology, 2, 136-178.
NMFS (1991) 56 FR 56812. Policy on applying the definition of species under the Endangered Species Act to Pacific salmon. Federal Register 56 No. 224 / November 20, 1991.
NMFS (1996) 61 FR 4722. Policy regarding the recognition of distinct vertebrate population segments under the Endangered Species Act. Federal Register 61 No. 26 / February 7, 1996.
NMFS (1997) 50 CFR Parts 222 and 227. Endangered and threatened species: Listing of several Evolutionary Significant Units (ESUs) of West Coast steelhead. Federal Register 62 No. 159 / August 18, 1997.
NMFS (2005) 50 CFR 226. Endangered and threatened species; designation of critical habitat for 12 evolutionarily significant units of West Coast salmon and steelhead in Washington, Oregon, and Idaho. Federal Register 70 No. 170 / September 2, 2005.
NMFS (2006a) 50 CFR 223 and 224. Endangered and threatened species: Final listing determinations for 10 Distinct Population Segments of West Coast steelhead. Federal Register 71 No. 3 / January 5, 2006.
NMFS (2006b) Endangered Species Act - section 7 formal consultation Biological Opinion and Magnuson-Stevens Fishery Conservation Act Essential Fish Habitat consultation for the operation and maintenance of the Lewiston Orchards Project. National Marine Fisheries Service, Seattle, WA.
NMFS (2010) Endangered Species Act - section 7 formal consultation Biological Opinion and Magnuson-Stevens Fishery Conservation Act Essential Fish Habitat consultation for the operation and maintenance of the Lewiston Orchards Project. National Marine Fisheries Service, Seattle, WA.
NMFS (2011) 5-Year review: summary & evaluation of Snake River sockeye, Snake River spring-summer Chinook, Snake River fall-run Chinook, and Snake River Basin steelhead. National Marine Fisheries Service, Portland, OR.
NRC. (1996) Upstream: salmon and society in the Pacific Northwest, National Research Counsil National Academy Press, Washington, DC.
Parmesan, C. & Yohe, G. (2003) A globally coherent fingerprint of climate change impacts across natural systems. Nature, 421, 37-42.
Poff, N. L. & Zimmerman, J. K. H. (2010) Ecological responses to altered flow regimes: a literature review to inform the science and management of environmental flows. Freshwater Biology, 55, 194-205.
Poole, G. C. & Berman, C. H. (2001) An ecological perspective on in-stream temperature: Natural heat dynamics and mechanisms of human-caused thermal degradation. Environmental Management, 27, 787-802.
Quinn, T. P. (2005) The behavior and ecology of Pacific salmon and trout, American Fisheries Society in association with University of Washington Press, Bethesda MD.
Richardson, S. & Rasmussen, L. (2007) Strategy for the ecological restoration of Lapwai Creek Watershed. Nez Perce Tribe, Lapwai, ID.
Rosenzweig, M. L. (1981) A theory of habitat selection. Ecology, 62, 327-335.
Sinclair, A. R. E. & Pech, R. P. (1996) Density dependence, stochasticity, compensation and predator regulation. Oikos, 75, 164-173.
Stanford, J. A. & Ward, J. V. (2001) Revisiting the serial discontinuity concept. Regulated Rivers: Research and Management, 17, 303-310.
Thomas, C. D., Cameron, A., Green, R. E., Bakkenes, M., Beaumont, L. J., Collingham, Y. C., Erasmus, B. F. N., deSiqueira, M. F., Grainger, A., Hannah, L., Hughes, L., Huntley, B., van Jaarsfeld, A. S., Midgley, G. F., Miles, L., Ortega-Huerta, M. A., Peterson, A. T., Phillips, O. L. & Williams, S. E. (2004) Extinction risk from climate change. Nature, 427, 145-148.
Turchin, P. (1995) Population regulation: old arguments and a new synthesis. Population dynamics: new approaches and synthesis (eds N. Cappucino & P. W. Price), pp. 19-39. Academic Press, London.
Vitousek, P. M. (1992) Global environmental change: an introduction. Annual Review of Ecology and Systematics, 23, 1-14.
Vitousek, P. M. (1994) Beyond global warming: ecology and global change. Ecology, 75, 1861-1876.
Williams, A. (2011) Floodplain delineation methodology utilizing LiDAR data with attention to urban effects, climate change, and habitat connectivity in Lapwai Creek, Idaho. Master Thesis, University of Idaho, Moscow, ID.
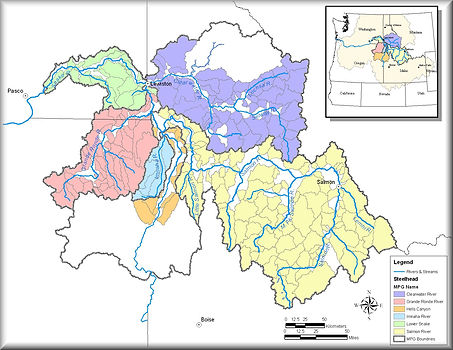
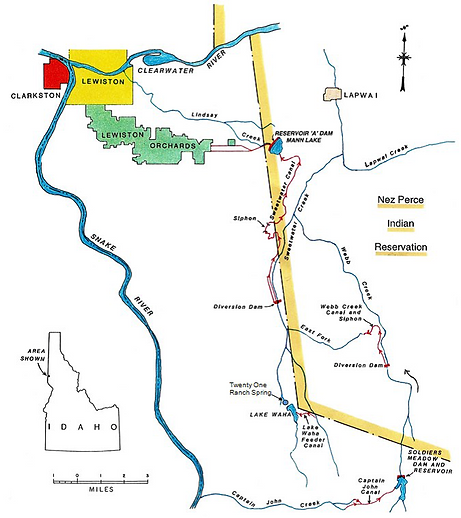